Results: Repressive
We ran a plate reader experiment of our aabb20 switch with increasing amounts of trigger a*b* and the long trigger a*a*b*b* which was to serve as a non-cooperative control. Our expectation was that increasing amounts of trigger should cause a decrease in fluorescence as the triggers should staple the switch shut bringing the switch and fluorophore together.
This however gave unexpected results. Whilst the fluorescence decreased initially for the long trigger, from 0nM to 50nM (equimolar to our switch), the fluorescence then increased. The fluorescence for the a*b* trigger did not appear to decrease at all:
​
​
​
​
​
​
​
​
​
​
​
We ran the acdb20 switch with our 5’ fluorophore strand for tracking on a polyacrylamide gels with a*b*, c*d* and c*a*b*d* triggers. Most samples were just mixed at room temperature whilst some were run after having been annealed (i.e. heated to 95°C then cooled so as to overcome any kinetic barriers).
​
​
​
​
​
​
​
​
​
​
​
​
​
​
​
​
​
​
​
​
​
​
​
​
​
​
​
​
The individual a*b*, c*d* and a*b*+c*d* samples (all equimolar to switch) did not show significant retardation. This suggests that the switch was not stapling shut. The shut structure with a*b* and c*d* triggers should be similar in shape and identical in mass to the same switch shut with c*a*b*d*. In addition, there appeared to be unbound fluorophore-labelled strand in these samples which is not seen in the switch and fluorophore alone.
​
​
​
​
​
​
​
We wondered if the results had something to do with the melting temperatures of individual a*, b*, c* and d* domains. The melting temperature for a b*a* strand (with the same domains as our a*b* trigger but binding straight rather a pseudoknot so able to be modelled by Nupack) has a melting temperature higher than room temperature. However, the melting temperature of individual a* and b* domains are very close to room temperature (IDT oligoanalyser). This may mean that they cannot stably bind by one domain, a likely intermediate to the stapled shut state.
​
​
​
​
​
​
​
​
​
​
​
​
We ran the same samples on a gel in the cold room (approximately 4°C) which should be less than the melting temperature of individual domains:
​
​
​
​
​
​
​
​
​
​
​
​
​
​
​
​
​
​
On this gel the switch appears to have been retarded slightly in the a*b* containing lanes and the amount of unbound fluorophore appears to have been reduced.
We ran the full sequence of our switch in Nupack with the sequence for a*b*. We had previously never done this with the full switch with flanking sequences as Nupack could not model our desired pseudoknot structure. This revealed a previously unconsidered structure with the a*b* trigger bound in the fluorophore binding 5’ flanking sequence through an accidental region of high complementarity in the b* domain:
​
​
​
​
​
​
​
​
​
​
​
​
​
If a*b* is present in large excess to the fluorophore-labelled oligo, as was the case in our plate reader experiment, then this binding mode could displace the fluorophore from the switch leading to unbound fluorophore. Unbound fluorophore will be more fluorescent than bound as it is not bound it the same molecule as the quencher. A similar weaker structure is also seen with the c*d* trigger. It is not clear why this weak binding is present on the gel in preference to the exact complementarity of the fluorophore-labelled oligo to this site.
Aim
Our overall aim for the repressive switches designs was to to demonstrate a cooperative dose-response to a trigger leading to closing of the switch thereby restricting access to the Ribosome Binding Site.
​
We began investigating this aim through similar steps to our activatory switches:
Can we assemble our switch as intended?
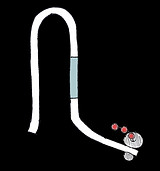
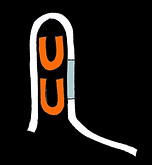
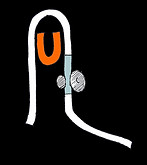
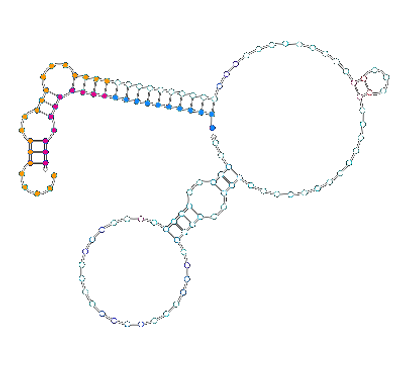
a*
b*
Fluorophore -labelled oligo binding sequence
aabb20 Switch
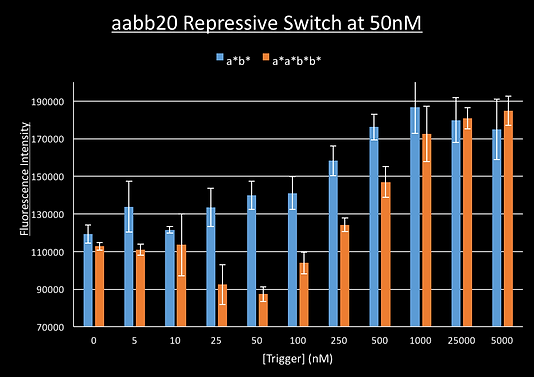
What other structures are possible?


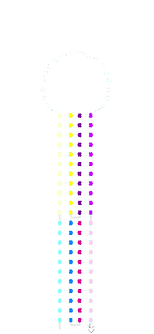
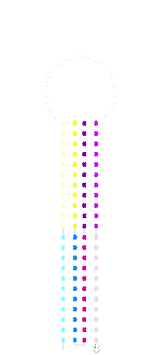
c*a*b*d*
a*b*
c*d*
Annealed
Annealed
acdb20 room-temp post-SYBR staining:
acdb20 room-temp
pre-SYBR staining:
Sw+F
Sw+F
Sw+F+c*a*b*d*
Sw+F
Sw+F+c*a*b*d*
Sw+a*b*+c*d*
Sw
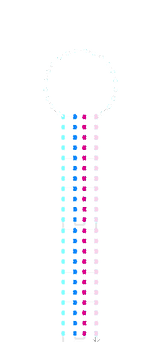
aabb20 Switch
a*b*
a*b*
Free Fl
Free Fl
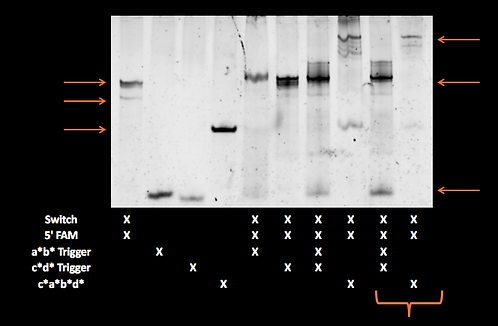
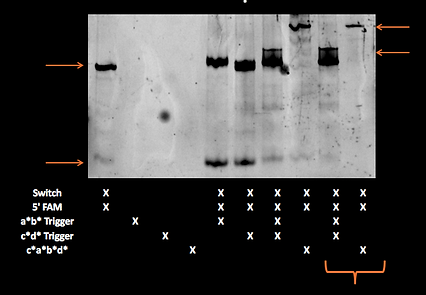
Annealed
Annealed
Sw+F+c*a*b*d*
F
Sw+F
Sw+F
Free F
Sw+F+a*b*+c*d*
Sw
Sw+F
Sw+F+c*a*b*d*
Free F
acdb20 cold-temp
post-SYBR staining:
Can we detect these structures via their melting temperatures?
Conclusion
Although unable to demonstrate cooperative switching via the mechanism we intended when we made our design we find it difficult to be disappointed in a repressive riboswitch design. The process of making these switches was a lot harder in the absence of the ability to model these sequences completely in Nupack. However when unexpected behaviour did arise the process of establishing and testing a hypothesis of the structures our switch formed was an interesting and informative process.
​
We believe that the lack of cooperative switching with our a*b* and c*d* triggers is best explained by the displacement of the fluorophore whilst the longer a*a*b*b* and a*c*d*b* triggers can form the structures depicted below:
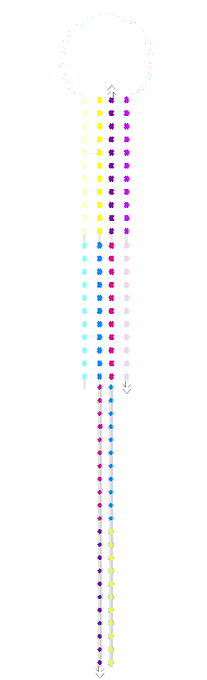
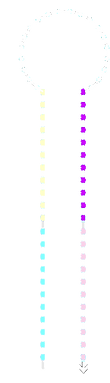
We felt that this explanation of fluorophore displacement however did not completely explain our plate reader results as the switch appeared to be stapled shut with c*a*b*d* without fluorophore displacement but fluorescence still increased when c*a*b*d* trigger concentration was high.
Looking at our repressive designs we remembered that another structure of the switch and long trigger was possible. This structure has two long triggers binding by two domains each instead of the desired structure where a single trigger binds by all four domains. Despite not modelling these switches in Nupack, the possibility of such incorrect binding had been discussed at an early design meeting but dismissed as unlikely as we felt that the intramolecular interaction between the two unbound domains of the trigger and their complementary domains in the switch would be faster and more favourable than the intermolecular interaction of a second trigger with those switch domains. This belief was justified by DNA origami were the staples are present in large excess to the circular ssDNA template to be folded yet structures with staple domains unbound do not appear.
​
​
​
​
​
​
​
​
​
​
​
​
​
​
​
​
​
​
​
​
​
​
​
​
​
​
​
​
​
​
​
​
The presence of dsDNA between the fluorophore and quencher-labelled strands on the switch would hold them further apart than in the single-trigger closed switch structure. It would also hold them further apart than in the switch structure without any trigger bound where the disordered DNA would be flexible enough for it to loop round so the fluorophore and quencher could be nearby for a proportion of the time. A change in structures first from disordered chain to stapled shut with equimolar c*a*b*d* or a*a*b*b* then to this double-trigger structure would therefore explain the dip in fluorescence around equimolar trigger:switch concentrations in our plate reader data.
We modelled this behaviour to see if it would explain the observed results.
Our supervisor Dr Jon Bath suggested we use thermal annealing to try to find these structures as it could be performed without our fluorophore-labelled oligo. Unlike the activatory switches which have relatively little difference in the amount of dsDNA in the open and unopened state (8bp as dsDNA in closed state vs 28bp in open state) our repressive designs should have no dsDNA without triggers and 44bp of dsDNA in a fully closed state. These should be distinguishable by their changes in fluorescence upon thermocycling with SYBR green.
aabb20 Switch
Single-trigger state
"Double-trigger state"
Extra domains unbound
We ran a thermal annealing program with three temperature spikes going from 25°C to 95°C then back to 25°C in 1°C/sec increments. At the end of every increment the fluorescence of SYBR green was measured three times then averaged. When there is more dsDNA SYBR green fluorescence is high.
​
​
​
For most DNA structures, segments going down in temperature should be identical to the slope going up and this was observed. This meant we could normalise all 6 segments and average them to give a smooth graph of their melting curve. We can then take the derivative by taking each data point and subtracting the value one degree below. This show the melting peaks.
​
​
​
​
​
​
​
​
​
​
​
​
​
​
​
​
​
​
​
​
​
​
​
​
​
​
​
​
​
​
​
​
​
For the switch alone, the a*b*, c*d* and a*b*+c*d* melting curves have a single point of inflection suggesting the sample has only a single structure present. Consistent with the idea of cooperative switching the presence of both triggers shifts the graph to the higher temperatures and makes it steeper.
The graph for the switch with the c*a*b*d* trigger shows two points of inflection around 72°C and 54°C.
To see if these correspond to the predicted single trigger and the double-trigger structures we set up another qPCR run where the ratio of switch to c*a*b*d* trigger was increased from 2:0 to 2:1 to 2:2, 2:4 and 2:8. If this is model is correct, we should see the 54°C peak increase in intensity as trigger concentration increases because the double-trigger state becomes would more significant part of the sample population.
​
​
​
​
​
​
​
​
​
​
​
​
​
​
​
​
​
​
​
​
​
​
​
​
​
​
​
​
​
​
​
​
​
​
​
As you can see the 54°C peak is most prominent in the sample with four times the concentration of c*a*b*d* than switch.
Similar results were obtained for the aabb20 switch with the a*a*b*b* trigger:
​
​
​
​
​
​
​
​
​
​
​
​
​
​
​
​
​
​
​
​
​
​
​
​
​
​
​
​
​
​
​
​
​
​
​
​
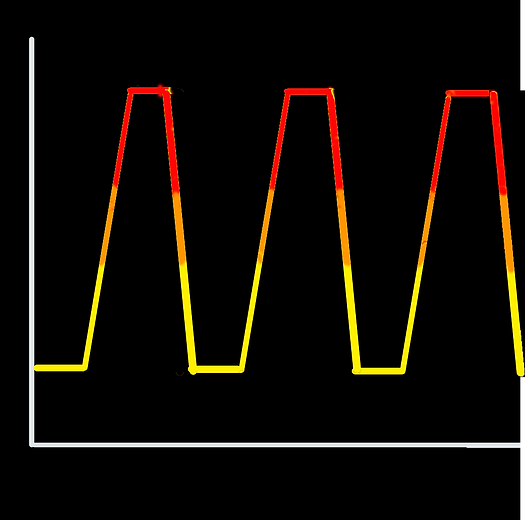
Temperature
Time
25°C
95°C
Change by 1°C every 12sec in heating/cooling stages
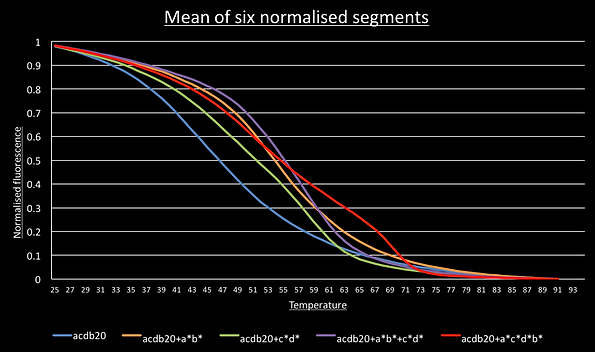
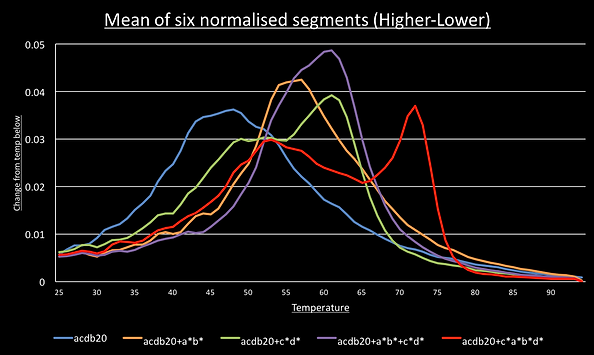

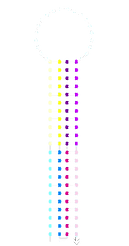

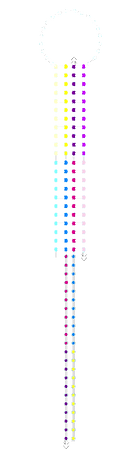
Predicted 72°C Tm
Predicted 54°C Tm
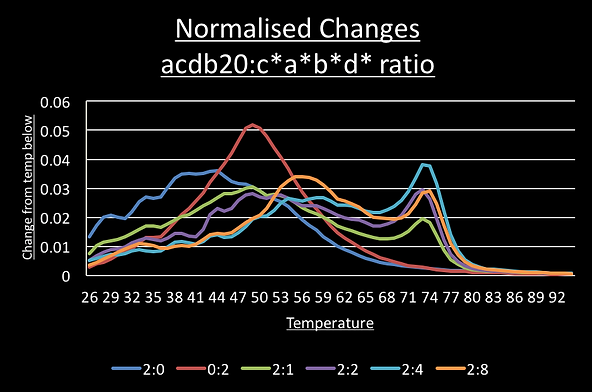
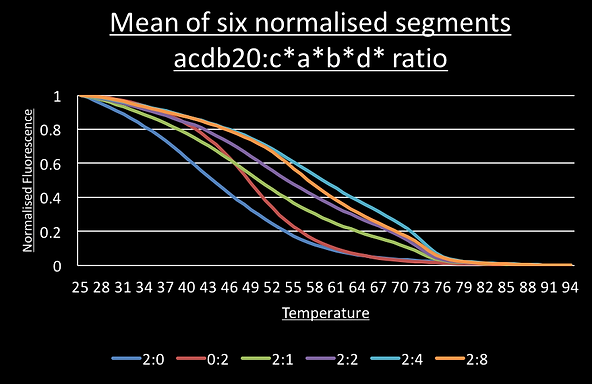
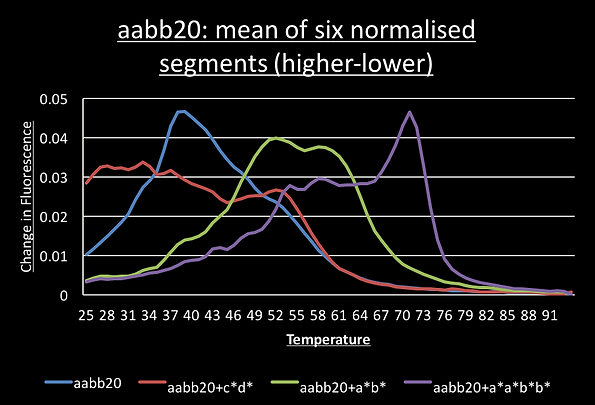
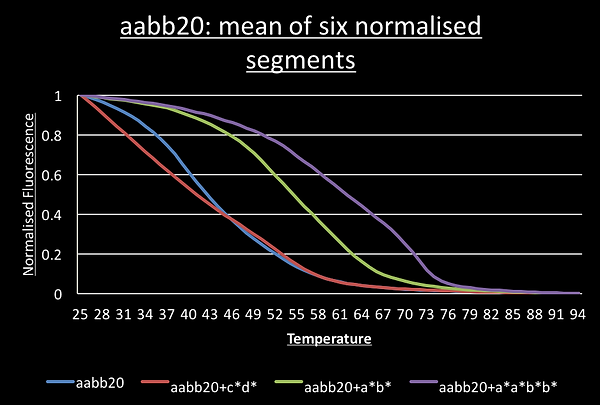
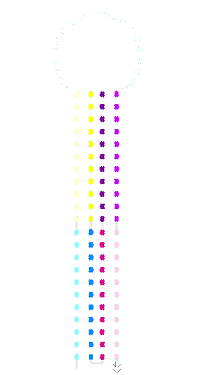

Other repressive riboswitch designs based upon the cooperative molecular receptors Plaxco et. al. are possible and these should be explored by future researchers as they have great potential in biological computing. We would suggest from our data lengthening the individual domains to change the melting temperature whilst trying to minimise non-specific binding.
acdb20 cold-room
pre-SYBR staining: